This research analysis presents an assessment of the Juragua nuclear
power plant, in Cienfuegos, Cuba, and its threat to the United States,
Latin America, the Caribbean, and Cuba itself, due to the safety problems
present in the design and construction of the reactors.
Over the past 20 years Cuba has been faced with an ongoing energy crisis.
Depending heavily upon imported oil, the Cuban government has attempted
to seek an alternative to oil through nuclear energy. In cooperation with
the former Soviet Union, Cuba embarked on a project to construct and operate
a nuclear power plant in Cienfuegos, known as Juragua. However, the collapse
of the Soviet Union halted construction at Juragua.
In 1976 Cuba and the Soviet Union signed an agreement to construct two
440 megawatt nuclear reactors in the south central province of Cienfuegos,
about 180 miles south of Key West, Florida. Juragua’s reactors are the
first Soviet designed reactors to be built in the Western Hemisphere in
a tropical environment. Actual construction of the reactors began in 1983.
The loss of Soviet subsidies to Cuba after 1990 has sent the Cuban economy
into decline. Also, the newly formed Russian Federation established new
economic ties with Cuba based on a market economy. As a result, on September
5, 1992, Cuba announced suspension of construction at Juragua due to Cuba’s
inability to meet the financial terms set by Russia to complete the reactors.
After 1995, bilateral cooperation between Cuba and Russia has
re-ignited the possibility of Juragua’s completion in the near future.
Recently, as of July 2000, an official from the Russian Federation announced
the intention to resume construction of Juragua. The United States views
a nuclear reactor in Cuba as a threat to its national security. The U.S.
has cited numerous safety concerns associated with Juragua, believing in
the event of an accident it would be exposed to radioactive fallout.
The possibility of an accident occurring at Juragua, upon its operation,
according to experts, is 15 times greater than the probabilities in a United
States plant. According to air weather patterns around Cienfuegos, it would
take only 24 hours for radioactive materials to reach Florida.
The report presents a comprehensive analysis of the Juragua plant, its
design and construction problems which presents a radioactive fallout threat
for Cuba, Latin America, the Caribbean, and the United States.
Energy resources are the various materials that contain energy in usable
quantities. These are present in any of the various energy forms that are
transformable to other forms, including electrical, mechanical, chemical,
and nuclear energy.
The main energy forms include chemical, hydro, nuclear, and geothermal
energy. Chemical energy includes such fuels as coal, oil, and natural gas.
Energy resources are usually classified in two general categories: renewable
resources and expendable resources. Renewable resources, such as water,
wind, solar, and tide, are replaced continuously by nature. Expendable
resources, such as oil and coal, are expended when used.
Energy may be classified as either primary energy, which is obtained
directly from nature, or secondary energy, which is energy derived from
primary energy. Primary energy sources include hydro, solar, wind, oil,
natural gas, and geothermal. Electrical energy is a form of secondary energy.
It is derived from primary energy by using thermal power plants to convert
heat energy and hydroelectric plants to transform the energy of water under
pressure.
Energy resources are being consumed at a high rate because of the growing
requirements of industries and the increasing demands of people. It has
been predicted that the world’s supply of fossil fuels will be used up
within a few hundred years.
The rate of consumption of oil has been rapidly growing. In many countries,
oil has been the primary fuel used for electricity generation. The fast
growth of oil consumption is due mainly to its use as fuel for automobiles
and airplanes and the fact that it is easier to recover and transform to
other energy forms than solid fuels.
The distribution of natural gas reserves is not accurately known. Estimates
of these reserves are approximate. In the United States, the use of natural
gas has risen consistently because of its relatively low cost. It has been
estimated that the world’s hydropower resources amount to twice the current
annual generation of all hydroelectric plants in the world. The available
hydropower depends on water inflows, and estimates of the available hydro
energy could be very approximate.
Geothermal energy is an almost limitless reserve. The temperature of
the Earth increases with depth in the Earth’s crust. Geothermal energy
has been used very limited to supply hot water or as geothermal power plants
of low consumption.
It has been estimated that the heat content of the world’s reserves
of uranium is more than 300 times the heat content of the world’s reserves
of fossil fuels. Using nuclear fission, current nuclear power plants convert
only a small fraction of the energy present in the nuclear fuel. However,
the unexpended fuel can be reprocessed for use in breeder reactors.
The oceans regularly rise and fall in response to the relative positions
of the Sun, Earth, and moon. The water elevation is not the same at different
places. The variation of water elevation can be used by electric power
plants to produce electric energy. However, such power plants are extremely
expensive, and the power output is quite variable.
Wind energy has been used in windmills around the world. Wind turbines
have also been used for electrical energy production in many countries,
but these have been in the low power levels. The Sun is the ultimate source
of energy because it has immense energy reserves. Solar energy is usually
used for space heating and water heating. Large collectors are necessary
to accumulate small amounts of power.
In 1878 Thomas A. Edison began work on the electric light and formulated
the concept of a centrally located power station with distributed lighting
serving a surrounding area. He perfected his light by October 1879, and
the opening of his historic Pearl Street Station in New City on September
4, 1882, marked the beginning of the electric utility industry.
At Pearl Street, dc generators, then called dynamos, were driven by
steam engines to supply an initial load of 30 kW for 110-V incandescent
lighting to 59 customers in a 1-square mile area. From this beginning in
1882 through 2000, the electric utility industry has grown at a remarkable
pace- a growth based on continuous reductions in the price of electricity
due primarily to technological accomplishment and creative engineering.
At present, two methods are commonly used for electric bulk-power generation.
Both methods employ an electric generator that converts the mechanical
energy of the prime mover to electrical energy. The main difference between
the two methods is the source of the mechanical energy used to rotate the
generator.
One method makes use of water under hydraulic pressure to provide the
mechanical energy to rotate a hydraulic turbine whose shaft is coupled
to the generator shaft. The efficiency of conversion of the energy available
from the water to electric energy is quite high- up to 80% to 90%.
The other method employs a boiler to convert the energy of the fuel
– coal, oil, or nuclear fuel-into heat energy, which is used to transform
water into high temperature steam at very high pressure. The steam
rotates the steam turbine in the same way as the water turns the hydraulic
turbine. The efficiency of conversion of the energy available from the
fuel to electric energy is much lower than the first method, typically
ranging from 30% to 40%.
The steam turbine generating units are the most widely used in the United
States to produce electricity. In a fossil-fueled steam turbine, the fuel
is burned in a boiler to produce steam. The resulting steam then turns
the turbine blades that turn the shaft of the generator to produce electricity.
In a nuclear-powered steam turbine, the boiler is replaced by a reactor
containing a core of nuclear fuel. Heat produced in the reactor by fission
of the uranium is used to make steam. The steam is then passed through
the turbine generator to produce electricity.
Steam turbine generating units are used primarily to serve the
base load of electric utilities. Fossil-fueled steam turbines generating
units range in size from 1 megawatt to more than 1,000 megawatts. The size
of nuclear powered steam turbine generating units ranges from 75 megawatts
to more than 1,400 megawatts.
Hydroelectric power is the result of a process in which flowing water
is used to spin a turbine connected to a generator. The two basic types
of hydroelectric systems are those based on: falling water; natural river
current. These conventional hydroelectric generating units range in size
from less than 1 megawatt to 700 megawatts. Because of their ability to
start quickly and make rapid changes in power output, hydroelectric generating
units are suitable for serving peak loads and providing spinning reserve
power, as well as serving base load requirements.
The ultimate objective of any power system is to deliver electrical
energy to the consumer safely, reliably, economically, and with good quality.
Operation of the power system requires that proper attention be given to
the safety not only of the utility personnel but also of the general public.
At the consumer load centers, electrical energy is converted to other more
desirable and useful forms of energy. This implies that the supply of electricity
should be available where, when, and in whatever amount the consumer requires.
Electrical loads are commonly grouped into four categories: residential,
commercial, industrial, and other. The residential loads are private homes
and apartments. They include lighting, cooking, heating and cooling, refrigerators,
water heaters, washers and dryers, and many other different appliances.
Commercial loads include office buildings, department stores, grocery stores,
and shops. Industrial loads consist of factories, manufacturing plants,
and other industrial factories. Industrial loads contain various types
and sizes of motors, fans, presses, furnaces, and so on.
Electrical load refers to the amount of electrical energy or electrical
power consumed by a particular device or by a whole community. It is also
referred to as electrical demand. At the individual consumer level, the
electrical demand is quite unpredictable. However, as the demands of the
various users are accumulated and added at a feeder or a substation, they
exhibit a definite pattern.
The U.S. electric power industry is organized to ensure that an adequate
supply of electricity is available to meet all demand requirements at any given
instant, both now and in the future. The rating of a generator is a measure
of its ability to produce electricity. The capacity of the generator is
the full load continuous rating of the generator under specified conditions.
Net capability is the steady hourly output that the generating unit is
expected to supply to the system load. The capacity of a generator is generally
higher than its net capability.
The generating units operated by an electric utility vary by intended
usage; that is, by the three major types of load-base, intermediate, and
peak-requirements the utility must meet. A base- load generating unit is
normally used to satisfy all or part of the minimum or base load of the
system and, as a consequence, produces electricity at an essentially constant
rate and runs continuously. Base-load units are the newest, largest, and
most efficient of the three types of units.
A peak-load generating unit, normally the least efficient of the three
unit types, is used to meet requirements during the periods of greatest
or peak load of the system. Intermediate-load generating units meet system
requirements that are greater than base load but less than peak load. Intermediate-load
units are used during the transition between base-load and peak load requirements.
Utilities also have reserve or standby generating units, which are available
to the system in the event of an unexpected increase in load or an unexpected
outage of the system.
From both an operational and functional point of view the power network
can be divided into several substructures based upon operating voltage
levels. Highest on the voltage scale is the transmission or the power grid.
The power is transformed in bulk power substations and fed into the sub-transmission
system. The sub-transmission system forms and intermediate and more fine
meshed link between the grid and the distribution circuits. The power finally
is fed into the fine meshes of the distribution system via distribution
substations
In the United States, the voltage of large generators is usually in
the range13.8 to 2.4kv (kilovolts). Large modern generators, however, are
built for voltages ranging from 18 to 24kV. However, there is no standard
for generator voltages. The voltage generated at a station is stepped up
to transmission levels in the range 115 to 765kV. The standard high voltages
are 115, 138, and 230kV. Extra high voltages are 345, 500, and 765kV. High
voltage transmission usually employs overhead lines supported by steel,
cement, or wood structures. The application of underground transmission
is mostly confined to urban areas and wide bodies of water. The power is
then stepped down depending on the use or application.
As of January, 1999, the existing capacity of U.S. electric utilities
totaled 686,692 megawatts. Based on primary energy source, coal-fired capacity
represented 44% (299,739 megawatts) of the nation’s existing capacity.
Gas-fired capacity accounted for 18%(125,386 megawatts); nuclear,
14%(97,070 megawatts);petroleum, 9%(62,959 megawatts); hydroelectric,11%
(77,593 megawatts; other, 11% (77,593 megawatts). Of the existing capacity,
conventional steam-electric units accounted for 62%. Nuclear units accounted
for 14%; hydroelectric,11%. The approximate existing capacity in the United
States is of 3,300 watts per person. Construction costs for a typical plant
range from $450 per kilowatt for combined-cycle technologies to $1,100
per kilowatt for coal steam technologies. Refurbishment of existing plants
is sometimes less expensive and a better option to consider.
Up to 1959, Cuba was supplied of electrical energy by the following
major utilities:
(1) Compañia Cubana de Electricidad (CCE). CCE was a subsidiary
of the American and Foreign Power Company, previously part of the Electric
Bond and Share Co.-EBASCO. CCE’s service territory included the Eastern
part of Pinar del Rio province, La Habana, Matanzas, Las Villas, Camaguey,
and the Southern part of the Oriente province.
(2) Hernandez y Hermanos. Its service territory
included the western part of Pinar del Rio province and the
cities of Trinidad, Casilda and several towns in Las
Villas province.
(3) Tabares. Its service territory
included the northern and central part of the Pinar del
Rio province
(4) Islas de Pinos utility. Covering Isla de Pinos
(5) Many other spotted areas were served by the large sugar
mill industry or the larger industrial complexes in the Island.
All these utilities were franchised and regulated by the Public Service
Commission, under the Ministry of Communications. Presently, all electric
generation, transmission, and distribution of electric energy in Cuba is
controlled by the government through the entity Empresa Electrica Cubana,
under the Ministry of Basic Industries. There are no private electric utility
companies in Cuba.
Cuba has an installed generating capacity of 3,500 megawatts. However,
the net generating capacity is only 1,200 megawatts. The industry employs
some 29,000 workers, of which, 4000 are technicians, and 850 are engineers.
The electrical energy demand was in 1996 of 2,500 megawatts, distributed
as follows: 60% industrial; 4% agricultural; 8% commercial; 25% residential.
The rest is for miscellaneous loads.
However, in 1999 the demand diminished to 950 megawatts, mainly due
to the large decrease in the industrial and residential loads.
The composition of the main units, equipment, instruments, and components
is very diverse. The main suppliers are: United States (pre Castro), former
Soviet Union, Japan, Italy, France, Czech Republic, Germany. The main generating
plants are: Mariel, capacity 600 megawatts; Tallapiedra, capacity 200 megawatts;
Regla, capacity 200 megawatts; Santa Cruz del Norte, capacity 300 megawatts;
Antonio Guiteras, capacity 350 megawatts; Cienfuegos, capacity 400 megawatts;
Felton, capacity 250 megawatts; Nuevitas, capacity 200 megawatts; Rente,
capacity 300 megawatts; Hanabanilla, capacity 45 megawatts.
There are a total of 46 operating units located in 20 different sites.
The transmission voltage is 110 kV and 220 kV. The country is mainly interconnected
with a 220kV grid. Transmission conductors are ACSR 150mm. Transmission
structures are concrete, metal, and H frame made of wood. Distribution
voltages are 4.16 kV and 13.8 kV, and the system operates at 60 Hz. Distribution
conductors are 150 mm, 70 mm, and 35 mm ACSR. Almost all distribution is
overhead, except some underground in La Habana.
Approximately 95% of the generating units in Cuba use No. 6 fuel oil.
A 4% use No. 2 fuel oil. Due to the wide range of unit ages and country
of origin present in the system, the sizes and operating parameters are
very diverse. The age range of the units vary from 8 years, the newest
one installed in Felton, to over 45 years for the ones present in Cuba
before 1959. Usually accepted economical and technical life for steam generating
units is 30 t0 35 years. An estimated 35% of the units installed in Cuba
are small, inefficient, and over the accepted range of operating life.`
Cuba consumed 13 million tons of oil in 1989, of which, 7 million tons,
or 40 million barrels were for the generation of electricity. In 1999,
Cuba consumed, for all needs, 6.3 million tons of oil. Of these, approximately
1.3 millions were domestic oil .Domestic oil is not suitable as a fuel
for the generating units because of the high content of sulphur, 9%, which
if used in the boilers, creates sulphuric acid, which corrodes the boilers.
The percentage of Cuba’s oil consumption is as follows: 50% imported
crude oil; 35% imported, refined; 15% domestic production crude oil. Cuba’s
domestic oil reserves are scant, so increasing domestic production is not
feasible.
Cuba has presently an installed capacity of 290 watts per person. However,
it has a net generating capacity of 100 watts per person. It is estimated
that a country, in order to sustain a stable economic and industrial growth
should have available at least 500 watts per person of net generating capacity.
The most prevalent problems with the electrical energy system in Cuba
are: (1) insufficient net generating capacity; (2) dependence on imported
oil as fuel; (3) lack of reliability; (4) inefficiency of the system; (5)
poor condition of the transmission and distribution system.
This analysis take us to the fundamental question. Was it necessary
to install a nuclear power generating plant in Cuba?
What is nuclear power?
Nuclear power taps the ultimate source of energy which powers the universe
and its myriads of stars like our Sun. Nuclear engineers deliberately arrange
to split certain atoms-this is called nuclear fission. When this happens,
some matter gets destroyed, liberating huge amounts of energy. This energy
mostly ends up as heat from which you can make steam to drive turbines
and generators (referred to in sections above), and make electricity in
power stations.
By careful design using material like uranium, engineers ensure that
neutrons collide with uranium atoms, breaking them apart into unequal size
halves. This yields energy and more neutrons and is called nuclear fission.
Repeat this, and you have even more neutrons. If the uranium is the right
type-uranium 235, a potent heat-releasing but controllable chain reaction
starts up. This is what powers reactors.
Reactors use a low grade of U-235 which can not sustain the atomic bomb
type reaction. This is why reactors contain tons of uranium, whereas a
bomb needs only a few kilograms. Because reactor grade uranium, most of
which is uranium 238 which is not fissile, contains only 1 to 2% U-235,
neutrons have to be slowed or they simply bounce off other uranium atoms.
Engineers slow down the neutrons with a moderator which increases the
likelihood of them smashing another U-235 atom to continue the reaction.
The moderator can be graphite or ordinary water, designated pressurized
Water reactors, PWRs, the most commonest reactor type around the world.
In PWRs, the water slows the neutrons and also cools the core. Powerful
pumps cycle the hot water out of the reactor core into enormous steam generators.
JURAGUA
As seen above, over the past 20 years Cuba has been faced with an ongoing
energy crisis. Depending heavily upon imported oil, the Cuban government
has attempted to seek an alternative to oil through nuclear energy. In
cooperation with the former Soviet Union, Cuba embarked on a project to
construct and operate a nuclear power plant in Cienfuegos, known as Juragua.
However, the collapse of the Soviet Union halted construction at Juragua.
Recent bilateral cooperation between Cuba and Russia has re-ignited the
possibility of Juragua’s completion in the near future. The United States
views a nuclear reactor in Cuba as a threat to its national security. The
U.S. has cited numerous safety concerns associated with Juragua, believing
in the event of an accident it would be exposed to radioactive fallout.
Description
In 1976 Cuba and the Soviet Union signed an agreement to construct two
440-megawatt nuclear power reactors in the south central province of Cienfuegos,
near Juragua, about 180 south of Key West, Florida. Juragua’s nuclear reactors
are of the model VVER-440, of Soviet design and are the first Soviet-designed
reactors to be built in the Western Hemisphere in a tropical environment.
The arrangement was aimed at alleviating Cuba’s dependency upon foreign
oil while bolstering its electricity capacity. The importation of oil has
drained Cuba of its sparse hard currency. At the same time the country’s
production of electricity has been fraught with difficulties. As of 1992
Cuban power plants have been working at only 47% of their capacity, leading
to frequent blackouts. This figure has fallen further due to the relative
decline in the Cuban economy since 1998. Upon completion, the first reactor,
Juragua #1, would generate approximately 15% of Cuba’s energy demands.
Actual construction of the reactors began in 1983. The Soviet Union
supplied a majority of the reactor parts, dispatched technicians to supervise
construction, and trained Cuban engineers to operate the reactors. According
to 1992 GAO report, Russia tentatively scheduled the first reactor to be
operational in late 1995. This was due in part to the Cubans constructing
the reactor lacking experience and with all critical work being performed
by Russians or under their supervision.
However, the breakup of the Soviet Union disrupted construction at Juragua.
The newly formed Russian Federation in conjunction with its transitioning
into a market economy established new economic ties with Cuba. Current
bilateral ties between Russia and Cuba, now, involve providing technical
assistance to Cuba on a commercial basis. At the same time the loss of
Soviet subsidies to Cuba after 1990 has sent the Cuban economy into decline.
As a result, on September 5, 1992, Cuba announced a suspension of construction
at Juragua due to Cuba’s inability to meet the financial terms set by Russia
to complete the reactors.
A September 1992 GAO report estimated that civil construction on the
first reactor ranged from 90% to 97% complete with only 37% of the reactor
equipment installed. About 25% of the civil construction on the second
reactor was completed with the status of the equipment unknown.
Cuban-Russian attempts to resume construction at Juragua took place
in October 1995. A high-level Russian delegation with full backing of the
government arrived in La Habana to conclude an agreement to complete construction.
To raise the $ 800 million dollars necessary to complete the reactors,
Russia and Cuba decided to form a syndicate with potential third parties.
Companies in Britain, Brazil, italy, Germany, and Russia expressed interest
in an economic association.
However, nothing concrete came out at that time. Cuba was rewarded with
a $50 million dollar grant loan from Russia for support work at Juragua.
Cuba now receives financial support for the Juragua plant from the International
Atomic Energy Agency (IAEA). The AIEA has provided nuclear technical assistance
in atomic energy development and in the application of isotopes and radiation.
The AIEA has provided from 1991 to 1996 about $680,000 to Cuba to develop
the ability to conduct a safety assessment of Juragua reactors, and in
preserving or “mothballing”the reactors while construction is suspended.
This assistance increased during 1997 to 1999. It is estimated that through
the last 20 years the IAEA has provided Cuba with some $14 million dollars.
We will dealt with this topic in a following section of the report.
Recent events have lead to the speculation of resumption of construction
in the near future. Recently, July 2000, an official from the Russian Federation
announced the intention to resume construction of Juragua. This will be
accomplished through an international consortium of countries, including
Russia. Upon resumption of construction, the Juragua first reactor is expected
to be operational within a 14 month timespan.
Impact
In the event of an accident during Juragua’s operation radioactivity
could leak from the plant. Such an accident would have a severely adverse
effect upon Cuba, United States, Mexico, Central America, and the Caribbean.
Once the reactors are operational, Cuba will have to develop plans to deal
with nuclear waste generated by the reactors. Currently there are no appropriate
sites to deposit nuclear waste in Cuba.
The Cuban government plans to dump waste in an area at sea level near
the Juragua plant. This would contaminate flora, fauna and Cuban population.
Cuba’s attempt to establish a nuclear power plant has been met with
substantial opposition in the United States and from environmental international
agencies. Several experts indicate that the Juragua reactor is inundated
with safety problems: structural defects in support structure in key reactor
components, integral reactor systems, including the reactor vessels, steam
generators and primary cooling pumps were exposed to highly corrosive tropical
sea weather, poor training and experience level of the Cuban personnel
who were trained on Soviet model reactors which are different from
Juragua, and that as many as 10% of 5,000 approved welds in key reactor
equipment were found to be defective. Four similar Juragua type reactors
(VVER-440) in East Germany were immediately shut down by West Germany upon
reunification. Similar plants in Hungary, Czechoslovakia and Bulgaria were
under inspection, shut down, or have received extensive modification. Refer
to map of Eastern Europe.
The plant instrumentation and controls, for example, reactor protection
systems and diagnostics are behind Western standards. The separation of
the plant safety systems, to help assure that an event in one system will
not interfere with operation of others, fire protection, and protection
for control-room operators are below Western standards.
The reactors have poor leak-tightness of confinement. There is
also an unknown quality of plant equipment and construction, due to lack
of documentation on design, manufacturing and construction, and reported
instances of poor quality materials being re-worked at plant sites. There
are also major variations in operating and emergency procedures, operator
training, and operational safety among plants using VVER-440.
The possibility of an accident occurring at Juragua, upon its operation,
according to experts, is 15 times greater than the probabilities in a United
States plant. Currently, Cuba lacks a comprehensive system to perform systematic
readings that monitor radioactivity to prevent potential accidents. According
to air weather patterns around Cienfuegos, it would take only 24 hours
for radioactive materials to reach South Florida.
Meltdowns
How can radioactivity be released from a nuclear power plant? The only
way that potentially large amounts of radioactivity could be released from
a nuclear plant is by melting of the fuel in the reactor core. The fuel
that is removed from a reactor after use and stored at the plant site also
contains considerable amounts of radioactivity. To melt the fuel requires
a failure in the cooling system or the occurrence of heat imbalance that
would allow the fuel to heat up to its melting point, about 5000 degrees
F.
It might seem that all that is required to prevent fuel from overheating
is to promptly stop, or shut down, the fission process at the first sign
of trouble. Although reactors have such fast shutdown systems, they alone
are not enough since the radioactivity decay of fission fragments in the
fuel continues to generate heat that must be removed even after the fission
process stops. Therefore, reactors should have redundant decay heat removal
systems. In addition, emergency core cooling systems should be provided
to cope with a series of potential accidents, caused by ruptures in, and
loss of coolant from, the normal cooling system.
There are two broad types of situations that might potentially lead
to a melting of the reactor core: the loss of coolant accident (LOCA) and
transients. In the event of a potential loss of coolant, the normal cooling
water would be lost from the cooling systems and core melting would be
prevented by the use of the emergency core cooling systems( ECCS). However,
melting could occur in a loss of coolant if the ECCS were to fail to operate.
The term transient refers to any one of a number of conditions which
could occur in a plant and would require the reactor to be shut down. Following
shut down, the decay heat removal systems would operate to keep the core
from overheating. Certain failures in either the shutdown or the decay
heat removal systems also have the potential to cause melting of the core.
The water in the reactor cooling systems is at a very high pressure
(between 50 to 100 times the pressure in a car tire) and if a rupture were
to occur in the pipes, pumps, valves, or vessels that contain it, then
a blowout would happen. The specific LOCA initiating events have been identified
as:
A. Large pipe breaks
B. Small to intermediate pipe breaks
C. Small pipe breaks
D. Large disruptive reactor vessel ruptures
E. Gross steam generator ruptures
F. Ruptures between systems that interface with the cooling system
Studies have indicated that a core meltdown in a large reactor would
likely lead to a failure of the containment. Therefore, the containment
integrity is very important.
Fuel melting accidents release more than 200 different radioactive substances,
of which, 54 are very dangerous. The Nuclear Regulatory Commission, NRC,
which oversees the United States’ nuclear power plants, says exposure should
not exceed 25 millirem per year, while the Environmental Protection Agency,
EPA, has set a standard of 15 millirem, with ground water levels not to
exceed 4 millirem.
Aroutine chest X-ray contains 6 millirem. Dosages above 30,000 millirem
are known to cause cancer, and levels of 400,000 millirem can cause death
in days. Another international unit used is the curie. For example, the
nuclear accident at Chernobyl, the worst nuclear accident to date, spewing
about 100 million Curies, or 4x10^18 becquerels, of radioactive material
into the environment. By contrast, the Three Mile Island released only
some 15 Curies.
Accidental Release of Radioactivity from Juragua
Radioactive pollutants released into the atmosphere will form a plume
that can be transported and dispersed by air currents, thus reaching areas
distant from the release location. It is therefore possible to construct
maps of a plume impact, average time of arrival, and relative plume concentration
from a single pollutant release, given the release location, meteorological
data, a transport and dispersion model, and a statistical analysis program
to determine useful and accurate results.
The release of radioactivity to the air from a nuclear power station
accident differs in at least one way from that produced by nuclear tests.
The radioactivity is injected near the ground and not at high altitude
as with tests. The two scavenging mechanisms that physically remove the
radioactivity from the air, namely, wet and dry fallout, are more effective
when a radioactive cloud is near the ground rather than at high altitudes.
Meteorological data are routinely generated from the National Oceanic
Atmospheric Administration (NOAA). The NOAA, the Meteorological Center
and the Air Resources Laboratory (ARL) have conducted experimental data
on air emanating from Cienfuegos, in one complete year. The geographic
domain in the analysis for a Cienfuegos release was a grid (95 km. Spacing)
including all of the U.S., Mexico, the western Atlantic, Gulf of Mexico,
and the Caribbean sea.
A release was assumed every 6 hours for the month, with the transport
following each release continuing for a duration of 5 days. This duration
was chosen so all plumes would approach or cross the geographic domain
boundaries. Simplified graphs and maps are presented in the next page.
The main feature of the probabilities is that shows relatively higher
values to the west and northwest of Cienfuegos. Relative concentrations
at Miami, Fl., and Houston, Texas are about the same during the Summer.
However, Miami and Tallahassee, Fl. show very high probability of impact
during the winter. Average time of plume arrival, as well as earliest time
of plume arrival show Miami with the highest in all seasons. Central America,
the Gulf of Mexico, and the Caribbean, show very high probabilities of
impact as Tallahassee and Houston. The average time it would take for radioactivity
from Cienfuegos to reach southern Florida is 48 hours. The shortest time
would be less than 24 hours.
VVER-440 reactors
A VVER-440 reactor is a pressurized water reactor developed from a reactor
design based on the first nuclear submarine reactors in the Soviet Union,
where de-mineralized light water is applied as both cooling agent and for
moderating the neutrons. The first version, VVER-440/230, was developed
in the 60’s, while the VVER-440/213 was introduced in the 80’s. It is a
Russian version of the Pressurized Water reactor (PWR). There are three
standard designs-two 6 loop-440 megawatt(the 230 and 213 models), and 4
loop-1000megawatt output designs. Re-fuelings are conducted with the plant
shutdown.
The reactor core in a VVER-440 reactor is 3 meters in diameter, has
a height of 2.5 meters, and is enclosed by a cylindrical pressure receptacle
of steel, of a diameter of 4.3 meters and a height of 11.8 meters. The
total weight is 200 tons. The reactor core contains 312 fuel assemblies
and 37 control assemblies. Each fuel assembly consists of 126 fuel pins,
which in turn consists of uranium-dioxide pellets. The content of 235U
in the fuel is replaced by new, non-irradiated fuel assemblies. The temperature
of the cooling water as it leaves the reactor is between 295 and 300 degrees
Celsius.
Each reactor coolant loop includes a steam generator and a reactor coolant
pump. The water passes through the inside of the tubes in the steam generator.
The reactor coolant pump circulates the water for cooling the reactor core.
The system is pressurized to 2200+ pounds per square inch by a pressurizer,
which is connected to one of the reactor coolant loops. Refer to schematics.
In the third schematic (model 230), the numbers indicate:
1. Reactor
2. Steam Generator
3. Main Circulation Pump
4. Refueling Machine
5. Cooling pond
6. Deaerator
7. Steam Turbine
8. Generator
9. Steam Pipelines
10. Cooling Water Pipelines
11. Transformer
In the fourth schematic (model 213), the numbers indicate:
1. Reactor pressure vessel
2. Steam generator
3. Refueling machine
4. Spent fuel pit
5. Confinement system
6. Make-up feedwater system
7. Protective cover
8. Confinement system
9. Sparging system
10. Check valves
11. Intake air unit
12. Turbine
13. Condenser
14. Turbine block
15. Feedwater tank with degasifier
16. Preheater
17. Turbine hall crane
18. Electrical instrumentation and control compartments
A major difference between western designed PWRs and the VVERs is that
the latter have horizontal steam generators. The older VVERs have isolation
valves in the reactor coolant loops and accident localization compartments.
Water passing on the outside of the steam generator tubes is heated and
converted to steam. Steam in the VVER design is not expected to be radioactive.
The VVER 440 design includes accident localization zones and a confinement
rather than a true containment.
The VVER-440 in Juragua belong to the “second generation” of the VVER
family. However, they do not meet western standards. They also have an
inadequacy of the upper portion of the reactor’s dome retention capability
to withstand only 7 pounds of pressure per square inch, given that normal
atmospheric pressure is 32 pounds per square inch and United States reactors
are designed to accommodate pressures of 50 pounds per square inch. Normal
air pressure at sea level, the level at which the plant is being constructed,
is 14.7 pounds per square inch. Therefore, the dome cannot survive when
exposed to the atmosphere.
The design of the Cuban reactor has many features in common with those
of the U.S., but there are several differences that could lead to significantly
different reactions in the event of a serious accident. For example, while
the Cuban reactor, like the U.S. PWRs, use water to cool the reactor core,
the Cuban reactor uses a different system for handling the steam pressure
that would be generated by a severe accident.
In the Cuban reactor, the steam is condensed so that pressure
is reduced in the containment structure. If, in the case of a severe accident,
the system for condensing the steam is bypassed and the steam reaches the
upper portion of the containment in pressures greater than the upper portion’s
designed pressure retention capability of 7 pounds per square inch. The
containment could be breached and a radioactive release could occur. In
contrast, U.S. PWRs are designed to accommodate pressures of about 50 pounds
per square inch throughout the containment structure.
Another main difference between the VVER-440 reactors and reactors of
Western type is the degree of safety containment surrounding the reactor
tank of the VVER-440.The airtight safety containment of Western power plant
encloses the reactor tank, the primary-and secondary circuits, as well
as the steam generators. At a possible leakage, the safety containments
will see that the radioactive steam does not escape to the surroundings.
At Western reactors, this safety containment is made of prestressed concrete.
Also, there are devices for cooling the steam to decrease the pressure.
The construction surrounding the reactor systems of the VVER-440 has a
volume too small to relieve the pressure arising should a breach occur
in pipes of more than 32 mm in diameter. The construction is fitted with
valves, which are released if the pressure gets too high.
Integral reactor systems, including the reactor vessel itself, six steam
generators, five primary coolant pumps, twelve isolation valves and more,
were stored outside for months, exposed to the highly corrosive tropical
sea air and weather. No nuclear reactor of Soviet design has ever been
constructed in a tropical climate.
The group of the world’s seven richest countries (G7) has concluded
that all reactors of the VVER-440 type must be shut down as soon possible,
as the reactors are upgraded to Western safety standards. Even the World
Bank emphasizes the serious defects of reactors of this type, rendering
any reconstruction unprofitable. From an economical point of view, the
World Bank claims nuclear power plant with reactors of the VVER-440 type
to be the most expensive energy alternative for the years to come.
There are eight VVER-440 reactors in operation in former Eastern Europe
and Russia. These are localized in Bulgaria, Slovakia, Russia. 6 additional
reactors of this type have formerly been in operation in, but are now shut
down. Four reactors were in operation at the nuclear power plant of Greifswald
in former DDR. The reactors were dismantled by the German authorities after
the reunion due to the lack of security at this type of reactor. There
are two reactors in Armenia, but they are temporarily shut down due to
their poor state.
On the construction side, the VVER-440 reactors deviate from safety
standards of Western reactors. IEAA performed in 1991 a safety analysis
of the 10 reactors in operation, and found 100 safety aspects connected
to the design and the operation of the plants. More than 60% of these aspects
are of great importance when safety is concerned.
The main problems concerning the design
of the reactor type is as following:
· Deficiencies in the construction concerning the limitation
of discharges to the surroundings in case of breaches in pipes of more
than 32 mm in the primary circuit
· Lack of safety containment surrounding the core
· Limited capacity of the cooling system
· Unsufficient “backup” of the cooling system and safety system
· Lack of distinction between control systems and safety precautions
concerning fire
· Obsolete control room technology
Neutron irradiation of the reactor tank, causing the steel to become
brittle, is a vital safety issue of the VVER-440 reactors. The proximity
of the fuel assemblies to the steel walls in the VVER-440 reactor tank,
causes higher neutron irradiation than in other types of reactors, and
the walls to become brittle at a higher pace than normal. The VVER-440
reactor tank is made up of welded rings. The welded seams are particularly
exposed to neutron irradiation. As a remedy, in some designs during the
late 80’s, the outermost assemblies were replaced with steel rods.
Light-water reactors are considered safer than the graphite-cooled model
that was in use in Chernobyl, Ukraine, site of the world’s worst nuclear
accident. But the Russian-designed VVER-440 light water reactors do not
meet the safety standards of Western nations. The design is considered
unsafe and should not be in operation.
International Atomic Energy Agency(IAEA)
Since 1958, the IAEA, in promoting the peaceful uses of nuclear energy,
has been providing nuclear technical assistance to its member states through
projects that supply equipment, expert services, and training. Currently,
more than 90 countries receive nuclear technical assistance, mostly through
over 1,000 projects in IAEA’s technical cooperation program.
The United States is a member of IAEA and its major financial contributor.
IAEA is providing nuclear technical assistance to Cuba in 10 program areas,
including general atomic energy development, the application of isotopes
and radiation in medicine, agriculture, and nuclear safety. Most of the
assistance, however, has been for Cuba’s partially constructed nuclear
power reactors.
IAEA spent about $12 million on nuclear assistance projects for Cuba
since 1963 through 1996. About 75% of the assistance Cuba received through
these projects consisted of equipment, radiation related instruments, and
laboratory equipment. The rest was in the area of general atomic energy
development. IAEA recently approved an additional $1.7 million for nuclear
technical assistance projects for Cuba for 1997 through 1999.
In addition, IAEA spent about $2.8 million on training Cuban nationals
and research contracts for Cuba. The United States contributes about 40%
of the total funds of the agency for such projects. IAEA is assisting Cuba
in developing the ability to conduct assessments of the nuclear power reactors
and in preserving or “mothballing” the reactors while construction
is suspended.
Nuclear Waste Disposal
The disposal of radioactive waste from nuclear power plants is a very
serious problem. Nuclear waste can be generally classified as either low
level radioactive waste or high level radioactive waste. Low level nuclear
waste usually includes material used to handle the highly radioactive parts
of nuclear reactors, like cooling water pipes and radiation suits, and
waste from medical procedures involving radioactive materials. Low level
waste is comparatively easy to dispose of.
High level radioactive waste is generally material from the core of
the nuclear reactor. Most of the radioactive isotopes in high level waste
emit large amounts of radiation and have extremely long half-lives, some
larger than 100,000 years, creating long time periods before the waste
will settle to safe levels of radioactivity. Radioactive wastes, being
highly toxic, can destroy or damage living cells, causing cancer and possibly
death depending on the quantity and length of exposure. In addition, radioactive
material can be mutagenic, thereby transmitting biological damage into
the future.
Every 12-24 months the reactor of a nuclear power plant is shut down
and the oldest fuel assemblies, which have released their energy but have
become intensely radioactive as a result of fission, are removed and replaced.
The fuel which has been consumed is known as “spent” nuclear fuel, SNF.
Spent nuclear fuel can be dissolved in a chemical process called “reprocessing”,
which is used to recover desired radionuclides.
If SNF is not reprocessed prior to disposal, it becomes the waste form
without further modification. The only commercial reprocessing facility
to operate in the United States closed in 1972. Since that time, no commercial
SNF has been reprocessed in the United States. Where are the wastes stored
now?
Today, most SNF is stored in water pools or above-ground in dry concrete
or steel canisters at more than 70 commercial nuclear-power reactor sites
across the nation. Also, waste is stored underground in steel tanks at
four Federal facilities in Idaho, Washington, South Carolina, and New York.
Plans are to store SNF at Yucca Mountain repository in Nevada.
All high level radioactive waste must end its journey in long term storage.
The waste must not be allowed to escape to the outside environment by any
foreseeable accident, malevolent action, or geological activity. This includes
accidental uncovering, removal by groups intending to use the radioactive
material in a harmful manner, leeching of the waste into the water supply,
and exposure from geological movement activity.
The extreme lethality of a freshly removed spent fuel bundle is such
that a person standing within a meter of it would die within an hour. The
hazards associated with transportation, in particular the possibility of
accidents, are very serious. Therefore, the minimization of handling and
transporting spent fuel is a desirable objective.
Areas currently being evaluated for storage of nuclear waste are space,
under the sea bed, and large stable geologic formations on land.
Long term storage on land seems to be the favorite of most countries. Cuba
has not serious plans at this point for the proper disposal of nuclear
waste, neither is technical or economical prepared to dispose safely of
the nuclear waste.
Caribbean Early Warning System (CREWS)
The Caribbean Radiation Early Warning System, CREWS, is designed in
response to the technical challenges presented by the proximity of the
Juragua nuclear reactors to the U.S. and other Caribbean neighbors. The
primary objectives of CREWS are: (1) create an early warning system that
provides actionable and timely data for emergency preparedness in the event
of a catastrophic radioactivity release, and (2) establish a high sensitivity
regional monitoring network capable of detecting small releases of radioactivity
that could be pre-cursors of potentially larger operational problems.
The components of the CREWS regional monitoring system are comprised
of field sensors, a central data analysis facility, a central measurement
facility, and a meteorological support group. The backbone of the system
is a network of airborne radioactivity monitoring stations that are capable
of meeting the early warning and high sensitivity objectives of the mission.
The primary functions are:
· Field sensors: Semi automatic sensors will collect, assay,
and transmit raw data.
· Central Data Analysis Facility: This facility houses the processing
and analysis capability to monitor the data from the field sensors.
· Central Measurement Facility: This facility is capable of
achieving detection sensitivities of the order of micro becquerels per
cubic meter.
· Meteorological Support Group: A link will be created with
an existing facility to provide meteorological support in the event of
a significant release of radioactivity.
Conclusions
1. Three of the VVER projects started by the former Soviet Union during
the late 70’s and early 80’s encountered serious problems that led to their
suspension. Of these three, two found solutions to their problems through
the technical and financial help of other countries. Only Juragua remains
uncompleted
2. The Cuban government is actively seeking financial and technical
assistance to finish Juragua. Feasibility studies indicate that $400 million
dollars are needed to finish the first reactor. Once construction is resumed,
a 14 month time span will be needed to make operational the reactor.
3. The civil construction of the first reactor is 97% complete. Approximately
40% of the reactor equipment is installed.
4. In the event of an accident during Juragua’s operation, radioactivity
could leak from the plant with an adverse effect upon Cuba, United States,
Mexico, Central America, and the Caribbean.
5. The release of radioactive to the air from a nuclear plant is more
effective than the one produced from nuclear tests. Meteorological data
conducted by the National Oceanic Atmospheric Administration, NOAA, and
the Air Resources Laboratory, ARL, show an early arrival of radioactivity
to Florida of less than 24 hours. The average time of arrival would be
48 hours. In 72 hours, areas affected will be Central America, Mexico,
the Caribbean, and most of the U.S. Eastern Seaboard.
6. The cities with a major impact in the U.S. will be Miami, Florida;
Houston, Texas, and Tallahassee, Florida.
7. The main problems concerning safety related to the Juragua’s
reactor are:
· Deficiencies in the construction concerning the limitation
of discharges to the surroundings in case of breaches in pipes of more
than 32 mm in the primary circuit.
· Lack of safety containment surrounding the core
· Limited capacity of the cooling system
· Insufficient backup of the cooling system and safety system
· Lack of distinction between control systems and safety precautions
concerning fire
· Obsolete control room technology
· Neutron irradiation of the reactor tank could cause the steel
to brittle
· Reactor dome has a retention capability to withstand only
7 pounds of pressure per square inch. Standard in the U.S. is 50 pounds
per square inch
· Unknown quality of plant equipment and construction
· Lack of documentation on design, manufacturing and construction
· Reported instances of poor quality materials being re-worked
at plant site.
· Reactor protection systems and diagnostic behind Western standards
· Separation of the plant safety systems, and protection for
control room operators are below Western standards
8. The possibility of an accident occurring at Juragua, upon its operation,
is estimated to be 15 times greater than the probabilities in a United
States plant.
9. Cuba lacks a comprehensive system to perform systematic readings
that monitor radioactivity to prevent potential accidents.
10. Six similar reactors of the Juragua type operating in Eastern Europe
were shut down due to the lack of security of the reactors.
11. The IEAA performed in 1991 a safety analysis of the 10 reactors
similar to the Juragua’s, remaining in operation, and found 100 safety
aspects connected to the design and the operation of the plants.
More than 60% of these aspects are of great importance, and are not acceptable
by Western standards.
12. The group of the world’s seven richest countries (G7) has concluded
that all reactors of the VVER-440 type must be shut down as soon as possible,
and the reactors should be upgraded to Western safety
standards. The World Bank emphasizes the serious defects of the reactors
of this type, rendering any reconstruction unprofitable.
13. Integral reactor systems-including the reactor vessel itself, six
steam generators, five primary coolant pumps, twelve isolation valves and more-were stored
outside for months, exposed to the highly corrosive tropical sea air and
weather. Also, no nuclear reactor of Soviet design has ever been constructed
in a tropical climate.
14. Cuba has not serious plans at this point for the proper disposal
of the nuclear waste generated in Juragua, once in operation. Cuba is not
technically or economically prepared to dispose safely of the nuclear waste
The Juragua nuclear plant should not be permitted to start operation
under the present design and construction deficiencies.
BIBLIOGRAPHY
1. Publications of the Center for Security Policy, No. 92-D-41
2. Report by U.S. Council of Energy
Awareness: “The VVER design is very different from Western
counterparts, and does not meet Western safety standards”,
April, 1992
3. Nuclear Energy Information Center, “Source Book on Soviet Designed
Nuclear power Plants”, 1996
4. The Natural Resources Defense Council, “Backgrounders: The Juragua
Nuclear Plant”, 1997
5. Fisher-Thompson, Jim, “Recent Film Reveals Flaws of Cuban Nuclear
Plant” U.S. Information Agency, August, 1995
6. “Nuclear Safety: Concerns with the Nuclear Power Reactors in Cuba”,
Statement of Keith O. Fultz, Assistant Comptroller General , Resources
Community, and Economic Development Division, United States General
Accounting Office, to the House of Representatives, Committee on
International Relations, Subcommittee on the Western Hemisphere,
August, 1995
7. International Atomic Energy Agency Information Circular, INFCIRC/537,
July 1997
8. International Atomic Energy Agency, “International Conference on
Strengthening Nuclear Safety in Eastern Europe”
9. Berger, Mikhail, “Cuba Hopes for Return of Absurd Economics”, 1996
10. Cereijo, Manuel, “Facing the Threat of Juragua”, June 1995
11. Dillion, Paul, “Nuclear Threat Looms Nearby”, November, 1997
12. Robinson, Roger. Cuba and the Juragua Nuclear Power Complex. Testimony
From a Hearing of the house International Relations Committee. Subcommittee
on the Western Hemisphere, August, 1995
13. U.S. Congress. General Accounting Office. Nuclear Safety: Concerns
about the Nuclear Power Reactors in Cuba. 101th
Cong. 2nd. Session RECD-92-262, 1992
14. Rohter, Larry. “ Cuban A-Plant worries U.S. over safety”, February,
1995
15. Fonticiella, Herminia; Arrondo, Raul; Fonticiella, L. “The Inherent
Danger of The Juragua Nuclear Power Plant”, May 1996
16. Thomas Nielsen; Nils Boehmer; “Kola Nuclear Power Plant”, July 2000
17. Russian Ministry of Security, Report No. 1344-G, July 1992
18. Murray, R. “Understanding Radioactive Wasre”, 4th Edition, Battelle
Memorial Institute, Ohio 1994
19. BEIR V., Committee on the Biological Effects of Ionization Radiations,
National Research Council, 1990
20. “Nuclear Energy’s Dilemma: Disposing of Radioactive Waste safely”,
Comptroller General of the United States, 1977
21. “Nuclear Safety”, GAO/RCED-97-72
22. Report DOE/EIA-0219, April 1999
23. CREWS Proposal, June 1999
24. Nuclear Waste Briefs, Winter 1996
25. Report # DOE/EIA-0383, December 1999
26. Inventory of Electric Utility Power Plants in the United States
1999 Executive Summary, EIA/DOE, 1999
27. TED Case Studies #469, August 1999
28. Nucleus, X aniversario SEAN, Comision de Energia Atomica de Cuba,
No.7 1989
29. Boletin Tecnico, Central Atomica de Juragua, Centro de Informacion
Cientifico Tecnica de la UPI, No.7, 1988
30. Boletin Tecnico, Vol. III, No. 1-2, Centro
de Informacion Cientifico Tecnica, Cuba, 1990
31. Personal conversations of the author with numerous
Cuban engineers, scientists, technicians, workers, and government officials,
1988-2000.
Este y otros excelentes artículos del mismo autor MANUEL CEREIJO
aparecen en la REVISTA GUARACABUYA con dirección electrónica de:
http://www.amigospais-guaracabuya.org
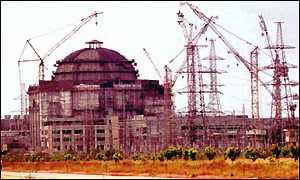
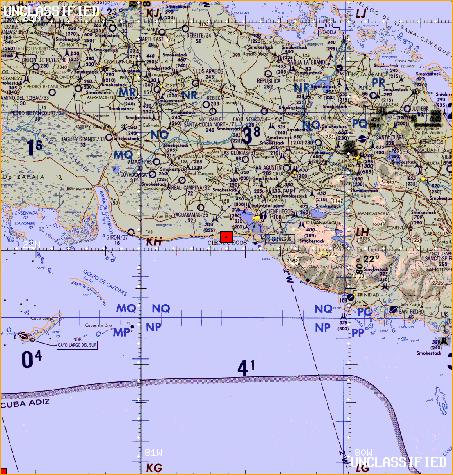
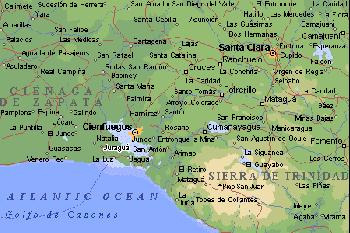
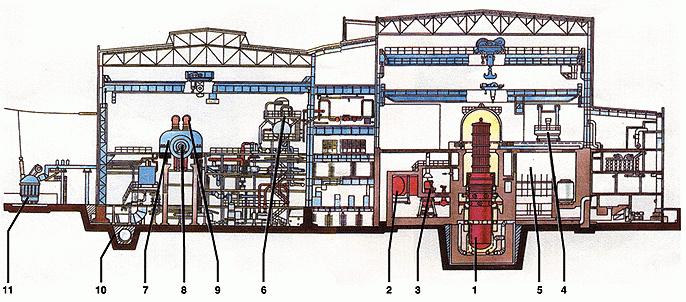
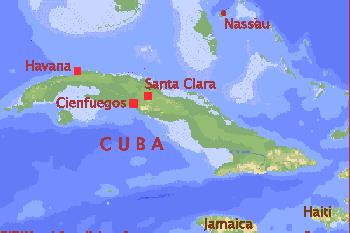
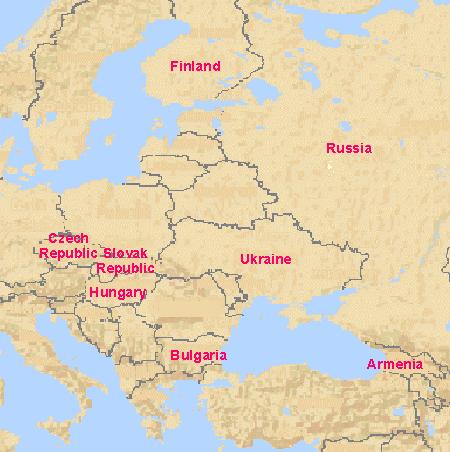
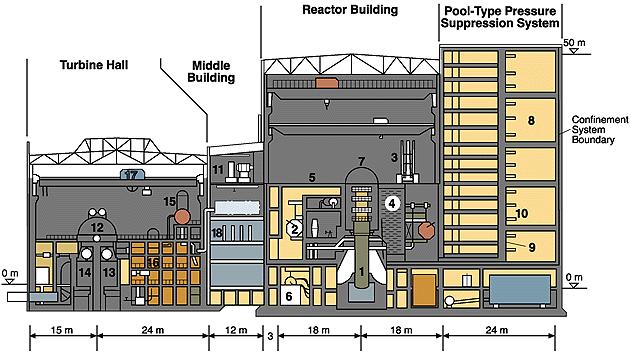
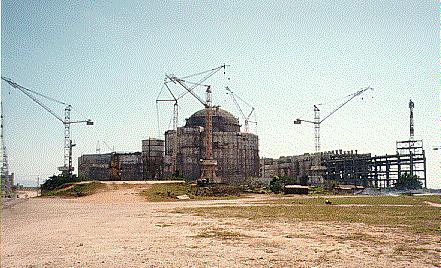
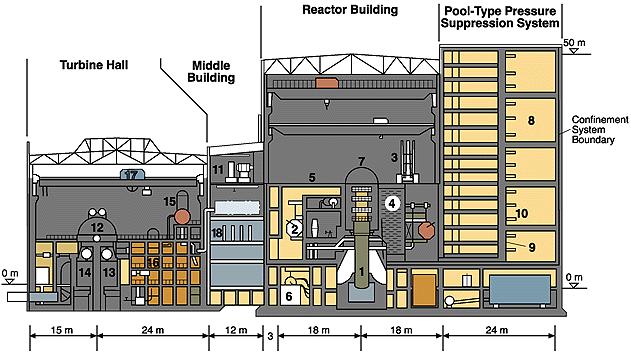
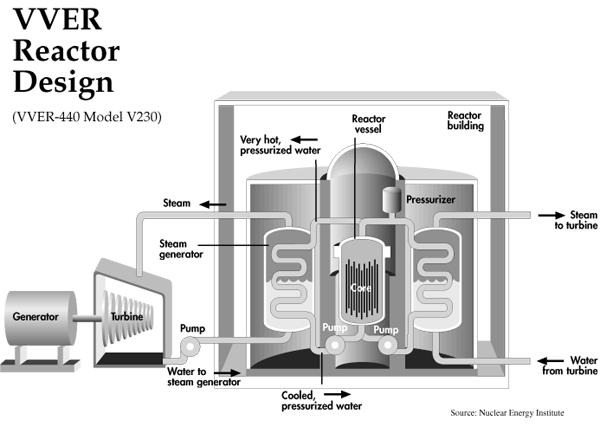
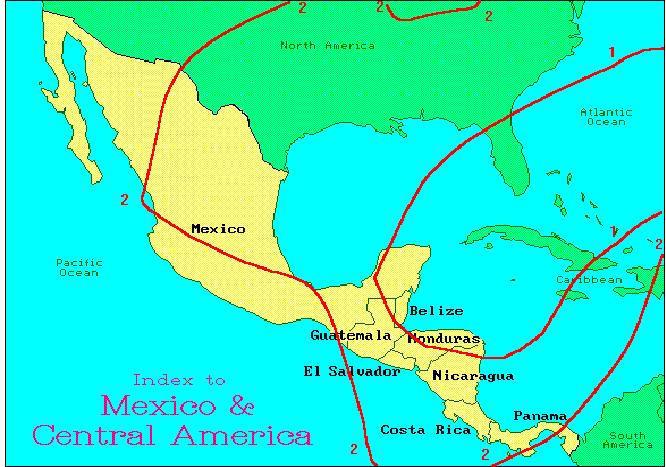
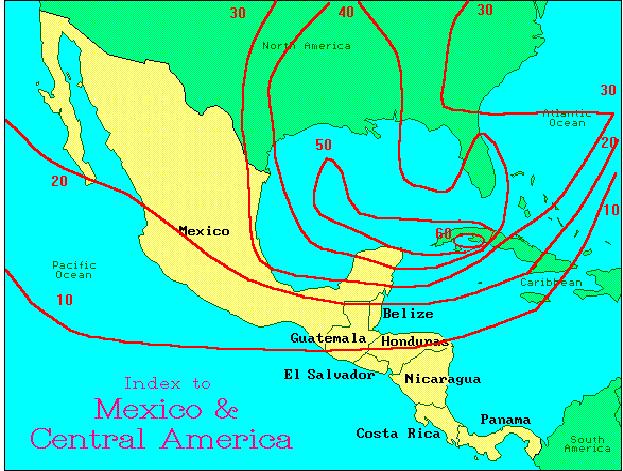
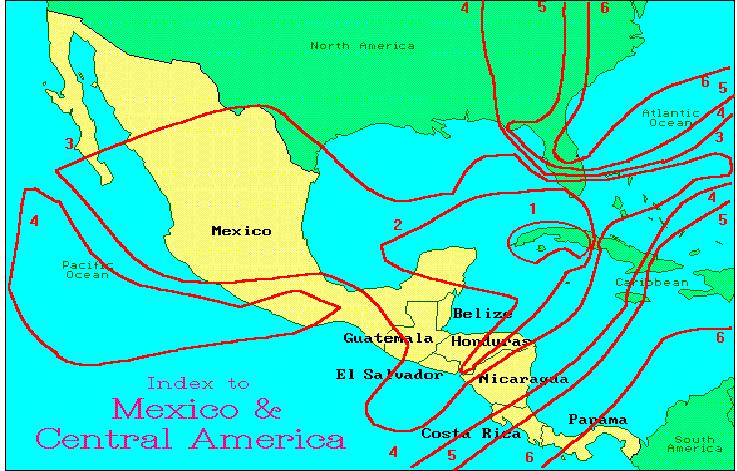
Earliest arrival, in days, of air from Cienfuegos, Cuba,
based on a year of trajectory calculations.
Percentage of time that air originating at Cienfuegos, Cuba
would override an area.
Average time, in days, for air to travel from Cienfuegos, Cuba
based on a year of trajectory calculations.